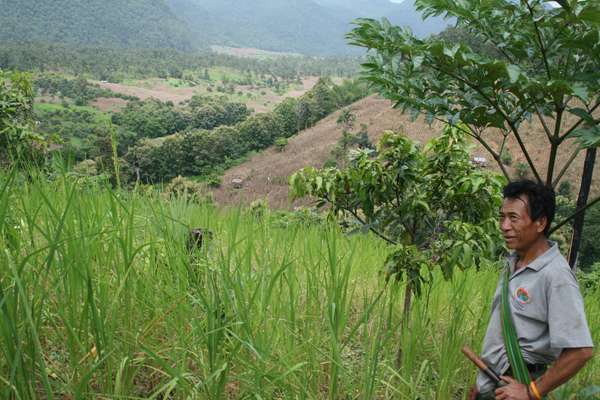
Figure 1. An example from Thailand of small-scale agricultural landscapes affected by interacting influences of climate and farmer management. Source: Tim Motis
ECHO recognizes climate change as a profound reality faced by small-scale farmers. Many of our publications have focused on helping farmers cope with related challenges such as heat and drought. Increasing farmer resilience and minimizing risk have been key elements of the practices we have written about over the years. We encourage “no regret” strategies, approaches that steward the land well and improve livelihoods, regardless of whether or not farmers face immediate changes to climate (Flanagan, 2015). However, we also view farmers as having an integral role in mitigating some of the driving forces of climate change—something we explore in this article, which is the first of a two-part series. Part 1 explores principles that are foundational to the strategies that we will present in Part 2, in the next issue of EDN.
What are farmers telling us?
Farmers and development practitioners often tell us of the adverse effects of changing climate that they experience in their communities. Patrick Trail, on staff with ECHO in Thailand, has a list of “five questions I ask every farmer.” One of those is, “As a farmer, what keeps you up at night?” or, asked in a different way, “What worries you most about the future of your farm and way of life?” Having visited about 150 farms in Southeast Asia, Patrick commented:
“I’d say that, as high as half the time, the answer is related to climate change...The most interesting observation I have made is that older farmers in rural areas, far removed and uneducated, very consistently talk about how seasonality has shifted. I often hear things like, ‘We used to know the exact week or window that the rains would begin, and we knew when to plant.’ ‘Now the rains might come early or late, may be sporadic, and may end early or flood late.’ These observations seem to indicate that climate is doing different things than it used to just 50 years ago.”
Why are farmers key to climate change solutions?
Agriculture significantly affects climate change
Climate change is a result of heat trapped in the atmosphere by the accumulation of “greenhouse gases” (GHG) emitted naturally and through human activity. These gases consist mainly of carbon dioxide (CO2), methane (CH4), and nitrous oxide (N2O). While agriculture is not the sole source of GHG, it does account for a large percentage of global emissions (24% according to EPA, 2020). Other human activities that generate these gases include the burning of fuels for electricity and heat, industry, and transportation.
Table 1 lists some agriculture-related contributors of GHG. Notice those that are associated with land degradation. Soil improvement, on the other hand, increases plant growth, which in turn keeps carbon (from CO2) on the land—in plant tissue and soil—instead of in the air. Later in this article, we highlight principles through which farmers can reduce GHG emissions from their lands and thereby participate in addressing climate change.
Table 1. Some agricultural contributors to emissions of agriculturally important greenhouse gases. | |
---|---|
Greenhouse gas | Contributors* |
Carbon dioxide | Deforestation and land clearing |
Soil degradation and loss | |
Burning of plant biomass | |
Methane | Digestion process of ruminant livestock |
Decomposition of organic matter in flooded rice fields | |
Decomposition of manure where oxygen is lacking, as often occurs with livestock managed in small spaces | |
Nitrous oxide | Denitrification, the biological conversion of nitrate (NO3-) to N2O, which occurs when oxygen is lacking |
Applying nitrogen fertilizers in excess of plant demand | |
*Contributors related to land degradation are highlighted in brown. |
Smallholders manage significant portions of land
Approximately 475 million households farm less than 2 ha of land in rural areas of economically poor countries (Lowder et al., 2016). Small farms (< 2 ha) occupy 12% of the world’s agricultural land. Within various regions, however, that percentage is higher. In Sub-Saharan Africa and South Asia, small farms occupy as much as 30% to 40% of agricultural land. Despite having few resources and facing difficult realities, these farmers produce food while making land-management decisions that impact much of the planet’s surface (Figure 1).
Land care necessitates farmer engagement
Farmer involvement, buy-in, and ownership are foundational to agricultural improvements in general. Initiatives to improve agricultural lands will not succeed unless farmers accept the practices being promoted. Farmers have an in-depth familiarity with their soils and livestock. Their knowledge, participation, and resources should be honored. These and related concepts are expanded on in ECHO summaries of information developed by Modernizing Extension and Advisory Services (MEAS).
What is carbon capture?
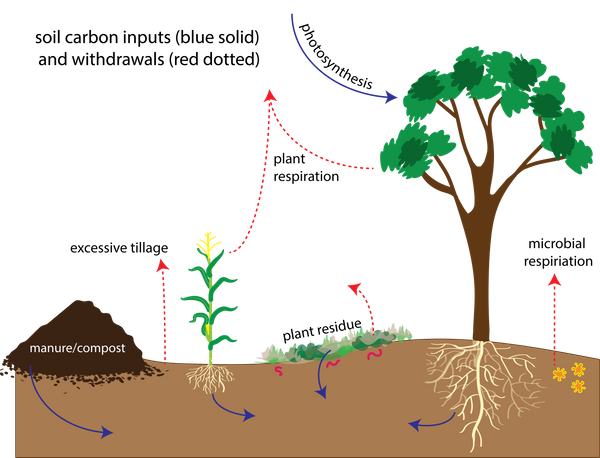
Figure 2. An illustration of carbon flow and resulting gains and losses of organic carbon in the soil. Source: Stacy Swartz
From an agricultural perspective, carbon capture (also called carbon sequestration) is the storage—on land—of CO2 removed from the atmosphere. The removal step relies mainly on photosynthesis, the process whereby plants use the sun’s energy to make carbohydrates—which contain carbon—from CO2 and water.
Carbon in plants moves into the soil in various ways (Figure 2). Roots release carbon-containing substances. Soil fungi called mycorrhizae obtain carbon from plant roots, while using their vast network of fungal threads to help plants gather nutrients and moisture. When plants and microbes die, some of the carbon in them is incorporated into soil organic matter. This happens as earthworms and other soil fauna transport surface residue into the soil, and as organic material is decomposed into stable forms (e.g., humus).
However, carbon in plants and soil can also move back into the atmosphere through decomposition and respiration. As soil microbes break down plant residue, they release CO2 into the air as they “breathe” or respire. Understanding that carbon does not stay in one place forever, the goal of carbon capture is to keep as much carbon on the land as possible, for as long as possible.
Why do soil carbon storage and land care matter?
Soil carbon is linked to the benefits of soil organic matter
Soil organic matter consists of plant or animal tissue in different stages of decomposition. Farmers reap numerous benefits from organic matter. Among them are a source of nitrogen, improved water infiltration, and greater retention of moisture and nutrients. These are important for small-scale farmers who have few options for fertility inputs, especially if they live in drought-prone areas. Organic matter is also rich in carbon, so any practice that increases organic carbon in the soil also increases soil organic matter, benefiting farmers.
Atmospheric carbon can be transferred to the soil
1"CO2 equivalent” is a metric unit of measure for the amount of CO2 equivalent to the warming potential of GHGs. As an example, 1 ton of CH4 equals 25 tons of CO2 equivalents, because the global warming potential of CH4 is 25 times that of CO2. N2O has 298 times more warming potential than CO2. CO2 is the most prevalent GHG and the one most easily addressed.
Globally, soils contain approximately 1500 gigatons (Gt; 1 Gt = 1 billion metric tons) of organic carbon. That amounts to more carbon than in the atmosphere (760 Gt) and in plants (560 Gt) combined (Lal, 2004; Paustian et al., 2019). Most of the world’s agricultural soils hold less carbon than they did before they were farmed, due to the clearing of vegetation for annual cropping. This presents farmers with an opportunity to increase the amount of carbon stored in their soils. For instance, conservation agriculture could sequester an estimated 9.4 to 13.4 Gt of CO2 equivalents1 by 2050 (Project Drawdown, 2020).
Soil protection keeps CO2 from being lost to the atmosphere
Minimizing soil tillage and erosion helps keep topsoil intact. One characteristic of healthy topsoil is the binding of individual soil particles into clumps called aggregates. Organic matter helps hold aggregates together, and aggregates protect the organic matter inside them (Six et al., 2002). When soil aggregates are broken apart and dispersed, the organic matter they contain is more susceptible to microbial breakdown and subsequent release of CO2. Land degradation often leads to depletion of soil organic matter. Conversely, restoring abandoned, degraded farmland could sequester an estimated 12 to 20 Gt of CO2 equivalents by 2050 (Project Drawdown, 2020). Farmers, of course, are crucial to making these lands productive again.
A few principles for carbon storage strategies in the tropics
An abundance of sunlight
The tropics are blessed with sunlight, which is necessary for photosynthesis. Think of photosynthesis as a freely-available resource that farmers harness with each square meter of land occupied by plants. Of course, sunlight is not the only requirement for photosynthesis; water is also critical. For example, cropping seasons in monsoonal areas are limited by the length of the rainy season. However, any practice that extends the growing season not only increases food production but also captures atmospheric carbon.
Rapid cycling of soil carbon
More direct sunlight in the tropics means they tend to have higher temperatures than temperate areas. Many parts of the tropics also receive high rainfall. The combination of heat and moisture favor rapid decomposition of organic material by soil microbes, which releases CO2 to the atmosphere. Moreover, some tropical soils are sandy or are comprised of clays with little capacity to form aggregates that would protect organic matter from microbial decomposition. These factors can make it difficult to build soil organic matter and to keep carbon losses from outpacing carbon gains.
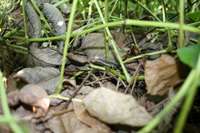
Figure 3. Plant-based mulch on the soil surface. Note the mixture of living/green and dead/brown material. Source: Tim Motis
Under these circumstances, maintaining plant matter on the soil surface is key (Figure 3). Research in no-till sugar cane fields in Brazil showed that soil carbon increases might have resulted even more from the maintenance of crop residues on the soil surface than from the lack of soil disturbance (Campos et al., 2011; Cherubin et al., 2018). Plant-based mulch protects the soil from intense heat, reducing carbon losses by slowing microbial respiration. At the same time, the mulch slowly releases nutrients that plants need. Keeping the soil covered with mulch mimics the blanket of leaves that is found in rainforests.
Limited availability of organic inputs
After crop harvest, many small-scale farmers need the residue (leaves, stalks) for livestock feed, as kindling to start cooking fires, or for other uses. In semiarid climates, the amount of plant biomass available for mulch is limited by low rainfall. Recognize these limitations when working with farmers to improve their soils. At the same time, look for creative ways to increase the availability of organic matter for soil improvement and, ultimately, soil carbon storage. A complete strategy to generate organic matter for soil enrichment could include one or more of the following techniques:
- Leave at least a portion of crop residues in fields, if at all possible.
- Take advantage of all sources of organic material, including animal manure and compost.
- Dig microcatchments, such as those used in the zai pit system (Motis et al., 2013), to concentrate fertility, harvest rainwater, and use available inputs as efficiently as possible. This approach is appropriate for dry climates.
- Integrate legumes or multipurpose trees and shrubs that can be pruned periodically (to minimize competition with crops for light, and to provide mulch). Lahmar et al. (2012) discuss an approach for the Sahel that combines zai holes (into which millet is planted) and native shrubs (Piliostigma reticulatum and Guiera senegalensis). Farmers prune native shrubs before the rainy season so that they do not shade the grain crop; the shrubs keep growing during the dry season and are among the last plants during the dry season to be grazed by livestock.
Non-uniformity of tropical and sub-tropical regions
Climatic conditions in the tropics vary. The tropics are often associated with heat and humidity, but there are also very dry regions, as well as highland areas that are quite cool. This means that strategies need to be appropriate to conditions and to farmer needs within the local context.
Concluding thoughts
We may never fully understand all of the factors that affect climate (nor the complex ways those factors interact). Yet the basic principles outlined in this article can help us move forward. The references section can be used as a starting point to deepen your understanding (top suggestions for further reading are marked with an asterisk [*]). We also encourage you to talk with farmers in your project area; their knowledge will be extremely valuable in developing effective, climate-smart strategies. In the next issue of EDN, we will review agricultural systems about which we have previously written and taught, this time addressing their potential to mitigate against climate change.
References
Campos, B.H.C., T.J.C. Amado, C. Bayer, R.S. Nicoloso, and J.E. Fiorin. 2011. Carbon stock and its compartments in a subtropical oxisol under long-term tillage and crop rotation systems. Revista Brasileira de Ciência do Solo 35: 805-817.
*Cherubin, M.R., D.M da S. Oliveira, B.J. Feigl, L.G. Pimentel, I.P. Lisboa, M.R. Gmach, , … C.C. Cerri. 2018. Crop residue harvest for bioenergy production and its implications on soil functioning and plant growth: A review. Scientia Agricola 75(3): 255–272.
EPA. 2020. https://www.epa.gov/ghgemissions/global-greenhouse-gas-emissions-data. Accessed 3 June 2020.
*Flanagan, B. 2015. Climate change and the role of development workers in helping rural agriculture communities adapt. ECHO Summary of MEAS Brief #3.
Lal, R. 2004. Soil carbon sequestration impacts on global climate change and food security. Science. 304:1623–7.
Lahmar, R., B.A. Bationo, N. Dan Lamso, Y. Guéro, and P. Tittonell. 2012. Tailoring conservation agriculture technologies to West Africa semi-arid zones: Building on traditional local practices for soil restoration. Field Crops Research 132:158-167.
*Lowder, S.K., J. Skoet, and T. Raney. 2016. The number, size, and distribution of farms, smallholder farms, and family farms worldwide. World Development 87: 16-29.
Motis, T., C. D’Aiuto, and B. Lingbeek. 2013. Zai pit system. ECHO Technical Note no. 78.
*Paustian, K., E. Larson, J. Kent, E. Marx and A. Swan. 2019. Soil C sequestration as a biological negative emission strategy. Frontiers in Climate 1.
*Project Drawdown. 2020. http://drawdown.org. Accessed 14 July 2020. [NOTE: This website gives detailed information on numerous practical ways to reduce atmospheric carbon.]
Six, J., R.T. Conant, E.A. Paul, and K. Paustian. 2002. Stabilization mechanisms of soil organic matter: implications for C-saturation of soil. Plant and Soil 241: 155–176.
Cite as:
Motis, T. 2020. Farmer-Centered Climate Change Mitigation. ECHO Development Notes no. 148